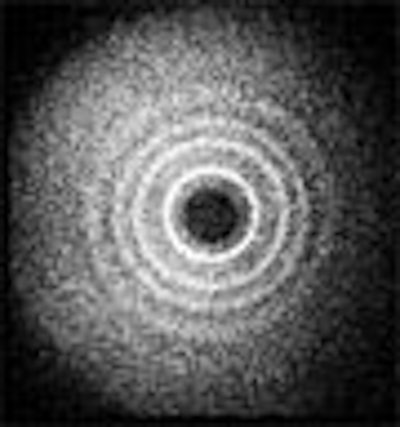
OSAKA - When Dr. Andrew Maidment arrived at the University of Pennsylvania in Philadelphia three years ago, it was an opportunity for him to rethink his strategy on breast cancer research, and look for the most promising applications ahead.
Given the chance to think big, Maidment saw that the thrust of research was getting smaller -- from molecules down to the very atoms inside them. That much was obvious: How else to diagnose and treat disease at an earlier stage? Of course, nuclear medicine has been thinking small for decades in pursuit of the same goals, while radiologists have often ignored the small potential in their big modalities.
A generation ago, the book The Powers of Ten (Morrison) covered the wonders that could be seen by viewing a human at 45 different orders of magnitude, Maidment said in a keynote address at the Computer Assisted Radiology and Surgery (CARS) meeting.
The job of imaging is to look at the smaller end of these magnitudes, or as Maidment put it, "from the organ level at 10 cm, down to the level of the tumor at 1 mm, down to the limits of conventional cross-sectional imaging at 1 mm, and finally to the structures of the cell to the genetic material in the cell, to the molecular composition of that material, and finally the level of the atom."
It's a world view that imaging professionals would do well to incorporate into their practices, said Maidment, who is an assistant professor of radiology and chief of the physics section of the department of radiology at the University of Pennsylvania.
"Perversely, we image the body to detect and diagnose diseases in the body on a large scale, using phenomenon that occur at a small scale," Maidment said. "We need to find ways to detect and diagnose disease earlier, and to determine prognosis -- something we really don't do today. And we need to do that by examining the physics, the biology, and the chemistry of the body at all scales."
And imaging still aims big though its physical basis is small. Radiography is based on atomic phenomena, and MRI and nuclear medicine are based on nuclear phenomena, he said.
What the big view has accomplished is, of course, phenomenal. Whole-body imaging and projects such as the Visible Human Project have enhanced our abilities in metastatic cancer surveillance. Even whole-body MR imaging is being conducted using massively parallel phased-array coils. Heart imaging, virtual colonoscopy, and even the whole-body screening of asymptomatic patients can be performed with multislice CT, Maidment said.
"The problem is we cannot distinguish between quiescent and active disease, and many of the diseases that we would find are not fatal," he said. For example, up to 6% of the autopsied bodies in the U.S. -- up to 20% in Japan -- are found to have thyroid cancer. But the patients didn't die from that disease, they died with it. So an important goal of imaging has to be an assessment of the biological significance of cancers. That's why prognosis is a key to moving forward, he said.
So the focus of 20th century medicine, radiography, MRI, and CT provides an anatomic window into the body, but organ morphology is often insufficient, though both nuclear medicine and fluoroscopy provide functional information. Medical imaging is quantum-limited -- not enough strength or signal to see the pathology -- and anatomy-limited, or hidden by other anatomy, as well as artifact- and observer-limited -- by pathologies it detects that are missed by the radiologist, Maidment said.
Breast imaging is a good example of this limitation. In 1993 when Maidment finished his doctoral thesis on digital mammography, he and many of his colleagues "believed that digital mammography would revolutionize breast imaging," finally making breast cancers obvious, he said. Yet it turned out that the overlying structure of the normal tissue on cancers is the principal limitation, even in digital mammography. This motivated his present focus on breast tomosynthesis -- the idea that you can take multiple views of the breast, then reconstruct planes within the breast.
With breast tomosynthesis, "you can see the spiculated nature of the lesion; it is clearly a cancer," he said.
The modality has value in clearing up cases that look like they might be a cancer on mammography, but actually result from the superimposition of normal tissues, thereby reducing the incidence of false positives. The injection of contrast extends its reach even further.
An additional limitation of imaging is a lack of contrast, as in the case of a toddler who couldn't bear weight on his right leg, Maidment said. Radiography was inconclusive due to a lack of contrast but scintigraphy two days later found a "toddler's fracture."
As a result, the future of traditional radiography may well be limited to dose-sensitive applications, he said. Further advances and inroads are likely to be found in 3D CT imaging applications, imaging methods that yield intrinsically higher contrast as well as methods that suppress normal structures and therefore increase conspicuity.
At the 1 cm, or tumor level of visualization, image fusion is going to be an important part of the equation, Maidment said, inasmuch as functional imaging needs an anatomic anchor. For this, integrated PET/CT is today's poster child, offering motion-free functional and anatomic information in a simultaneous acquisition by two scanners under the hood.
"There is a synthesis by combining PET and CT," he said. "This synthesis does not always exist with other techniques, so you're unlikely to find ... a PET/ultrasound or an MR/ultrasound system." But breast tomosynthesis combined with ultrasound is a distinct possibility, he said.
"At the level of 1 cm, data fusion and image registration are necessary for the relation of function and anatomic images," Maidment said. "But we need a consistent image acquisition geometry, and really to do this properly we need models of patient positioning and differences in motion."
"We're approaching the limits of cross-sectional imaging," he said. Most of today's cross-sectional modalities have resolution of about a half a millimeter, but are evolving. MRI is increasing field strength, improving gradient performance to current levels of 40-50 mtesla/m, increasing the number of radiofrequency (RF) channels to 76, and improving coil design, offering spatial resolution at the level of 150 mm, along with significant improvements in temporal resolution and signal-to-noise ratios.
![]() |
View from the Osaka International Convention Center. |
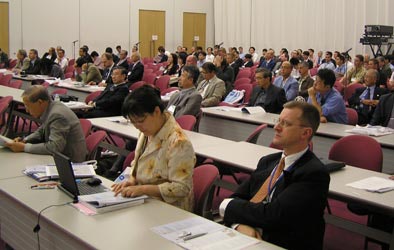
Dr. Andrew Maidment (lower right) and delegates attend CARS opening sessions. |
But if the signal does not exist, a better magnet can't help, which begs the development of new methods -- that are often based on traditional modalities. For example, today MR perfusion has replaced radioactive water studies of the brain, a different way of imaging MRI, Maidment said.
MRI of polarized helium imaging of the lung, as seen in a recent study of pigs, is opening up a trove of functional information about the lungs, which were a "black hole" until just a few years ago.
Another new development from an old modality is MR diffusion tensor imaging. "We use an RF pulse to excite protons and look at how they diffuse, and if there are structures in the body that limit their diffusion.... If there is some underlying structure, they will diffuse along that structure, and we can characterize that by an ellipse which describes the diffusion," he said.
The technology is being used to spectacular effect in neural imaging, in which diffusion tractography connects the various diffusion vectors along tracks, which correspond very closely to what is known about the wiring of the brain. The technique enables no less than the differentiation of neural anatomy at the level of interconnectivity of the neurons, Maidment said, and as a result, the ability to look at diseases that affect the wiring of the brain but not gross neural anatomy.
Animals to humans and back
At the cellular level, small animal imaging will guide us. The University of Pennsylvania's small animal imaging center ties radiology to nearly every other scientific pursuit at the university, Maidment said.
A micro-CT scanner images down to the level of the trabecular structure of a rat. A micro-PET scanner looks at functional imaging in small animals, recently demonstrating a mouse model of Parkinson's disease, he said.
Injection of the neurotoxin 6-hydroxydopamine into the mouse brain produced selective destruction of catecholamine (adrenalin, noradrenalin, and dopamine) neurotransmitter neurons. PET imaging for dopamine showed its absence in the treated region. Along with behavioral changes, PET in small animals can be used to study the neurodegenerative disease process associated with Parkinson's.
"You get similar results in SPECT," Maidment said. "And the nice thing about SPECT in small animals is that you actually get higher spatial resolution (than PET). So although SPECT is coming out of vogue in human imaging, it is becoming more prevalent in animal imaging."
One thing not yet performed in humans is optical imaging, which is different than nuclear medicine because the targeted agent must be activated by the probe. The excitation pulse activates the agent injected into the binding site, such as fluorochromes within the cell, and the photons it produces are then detected, enabling visualization of the cell.
For example, in a mouse that had an ovarian tumor, a T-cell was administered that had an optical agent, Maidment explained. When the agent binds to the tumor, "you can see over time an increase in the binding, and an increase in the definition of the tumor model," he said.
"What can we learn? Well, obviously there are human techniques that can be applied in animals, and our job is taking animal techniques and applying them to humans," Maidment said. "Both are necessary for medical research, and both medical researchers and imaging researchers will reap the benefits."
The molecular view
Molecular imaging implies the visualization of normal and pathological processes with biochemical and pharmacologic probes. Ideally, he said, it should not disturb the process it is detecting.
The advantages of molecular imaging include detecting earlier disease states, enabling visualization of pathologies that never manifest themselves morphologically, Maidment said. Even when it does manifest itself structurally, morphological imaging can't distinguish between active and inactive disease.
FDG-PET imaging, for example, has potential applications in neurological disorders, cancer, inflammation, infection, myocardial viability, atherosclerosis, muscle dysfunction, and clot detection, to name a few. And many more radiotracers are being used or are in the development pipeline.
Among them, CU-64 has therapeutic potential. I-124, BR-76, and BR-77 are PET and therapeutic isotope nuclides applicable to a wide range of compounds. Y-86 can potentially be used for dosimetry prior to Y-90 therapy, and there are many more applications.
Combined with traditional modalities for anatomic localization, the effect will be to eventually shorten the period from detection to treatment down to hours and days rather than months, Maidment said.
Then there are nanoparticles, tiny polymeric colloidal particles loaded with therapeutic and/or imaging agents that are either dispersed in a polymer matrix or encapsulated in polymers to detect and treat disease, Maidment said. In cancer targeting with quantum dots, for example, tumors can be targeted passively by virtue of their leaky vasculature, or actively by the high-affinity binding of quantum-dot antibody conjugants to tumor antigens, according to one experiment.
"Clearly this is the road to the future," he said. "As imaging scientists, we must familiarize ourselves with this technology and become involved with the development of new imaging agents."
Other research uses targeted MR contrast agents, for example, or nanoparticle angiogenesis imaging. Among the newest methods in molecular imaging is gene tomography, which uses labeling of cDNA, RNase, and other molecules to localize the expression of specific genes. Originally an invasive technique, it has now been demonstrated with micro-PET in animals and PET in humans.
The expression of specific genes will enable early disease detection and characterization, prognostication, and observation of treatment response, Maidment said.
Maidment and his colleagues at the University of Pennsylvania's x-ray physics laboratory have conducted coherent scatter-imaging experiments, which use x-rays to quantitatively measure the radiographic properties of calcifications to assess their composition, and improve the diagnosis of breast cancer.
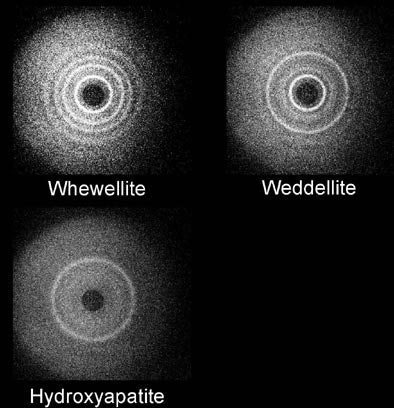
Coherent scatter system design prototype is based on a scanning pencil beam. The x-ray beam is filtered and collimated before hitting the target. The primary beam is attenuated by a beam stop. The radial distribution of the scattered x-rays is recorded on the detector. Above, whewellite, weddellite, and hydroxyapatite yield distinct scatter signatures. Images courtesy of Dr. Andrew Maidment. |
"The future of medical imaging, I believe, is tied to advances in other fields, and we can no longer content ourselves with strictly anatomy," Maidment said. "There's an increasing reliance today on image fusion, and we need to look at new ways of imaging specific diseases and specific metabolic pathways. We need to explore new agents and find new ways of ways of extracting information. Basically we need to improve lesion conspicuity."
By Eric Barnes
AuntMinnie.com staff writer
July 3, 2006
Related Reading
Image guidance evolves into imaging therapy, May 12, 2006
Imaging poised to transform the future of medicine, February 2, 2006
IMRT to 4D radiotherapy rely on QA for accuracy, May 4, 2006
NEMA paper touts imaging's role in oncology, April 6, 2006
CARS suite of the future sees key role for image-guided intervention, June 23, 2005
Copyright © 2006 AuntMinnie.com