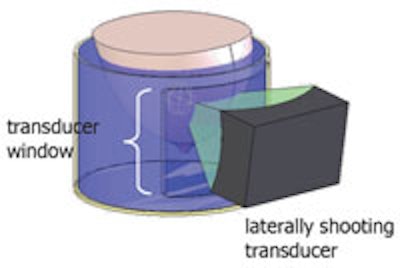
Clinical trials of MRgFUS for treating breast tumors are already under way. However, the geometry and tissue composition of the breast pose some unique challenges. So researchers at the University of Utah School of Medicine in Salt Lake City are working to develop an ultrasound treatment system specifically tailored for breast tumor ablation.
Allison Payne, PhD, a postdoctoral fellow at the Utah Center for Advanced Imaging Research (UCAIR), presented the group's latest results in October at the International Symposium on MR-guided Focused Ultrasound in Chantilly, VA.
Breast MRgFUS systems typically employ a geometry in which the patient lies prone with the ultrasound transducer located below the breast. While this setup can be effective in treating some locations, the presence of sensitive tissues makes it difficult to treat all lesions.
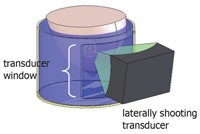
The Utah system, on the other hand, is designed such that the patient lies with the affected breast suspended in water in a cylindrical tank and the transducer is water-coupled laterally to the edge of the tank. This enables the transducer to rotate around the breast, providing about 270ยฐ of access and minimizing the volume of coupling fluid.
"By moving the transducer lateral to the breast, it not only avoids the ribs and nipple, but increases the acoustic window available to treat the lesion," Payne explained. "The transducer can rotate nearly 360ยฐ, allowing treatment of a greater fraction of breast lesions."
Payne and colleagues optimized the transducer design by performing acoustic and thermal simulations with constraints imposed by real patient breast data. For example, ablating lesions of up to 3 cm in a breast of up to 17 cm in diameter requires the transducer to offer electronic steering by up to 1.5 cm in all directions and have a radius of curvature of less than 15 cm. The low perfusion of breast tissue, meanwhile, suggests the use of a relatively low power density for lesion ablation. Grating-lobe clutter must be reduced to minimized normal tissue damage.
The ensuing transducer comprised 256 randomly placed array elements of 4 mm in diameter, with a 13-cm radius of curvature and an aperture size of 8 x 10 cm. The transducer has an operating frequency of 1 MHz and a power density of 2 W/cmยฒ, or 4 W/cmยฒ with active water cooling.
To test the transducer design, the researchers simulated thermal ablation of a 12-mm diameter circle. Twelve points were sonicated at 200 msec/pt, with 60 sec total sonication and an acoustic power of 31-52 W. The maximum temperature increase was approximately 21ยบ C in the focal plane. The thermal dose (a measure of equivalent heating time at 43ยบ C) accumulated after 60 sec of sonication was greater than 240 cumulative equivalent minutes (CEM) -- corresponding to fully necrosed tissue -- in all of the target area.
Payne explained that thermal dose -- basically an integral of temperature over time -- is used as a measure of tissue necrosis. "CEM in this particular case is cumulative equivalent minutes at 43ยบ C. Tissue is therefore necrosed after it has been raised to 43ยบ C for 240 minutes," she said. "Thermal dose is highly nonlinear, so once you get to about 57ยบ C, or 20ยบ C above body temperature, you can accumulate as much as 240 CEM every second."
MR monitoring
Optimization of the receiver coil used for MR imaging and temperature monitoring is equally important. Increasing the signal-to-noise ratio will enable faster, higher-resolution imaging of breast anatomy. In addition, higher signal-to-noise ratio means more accurate MR temperature mapping, resulting in improved thermal dose calculations and more efficacious treatment outcomes.
The researchers evaluated various types of RF receiver coil to maximize signal coverage and signal-to-noise ratios. They performed Matlab simulations to compare the performance of cylindrical ladder and circular loop arrays with that of a single chest coil, as generally used for MRgFUS. A 14-channel cylindrical ladder coil mounted directly on the cylindrical tank was seen to exhibit 2.7 times higher signal-to-noise ration than a single loop placed at the chest wall.
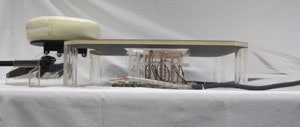
"The 14-channel ladder coil and a 26-channel circular loop coil both gave excellent signal-to-noise ratio over the area of interest," Payne said. "However, the ladder coil geometry is easier to adapt to the geometry of the cylinder that holds the breast. Also, more channels require more data storage and increases reconstruction time."
Ultimately, as a trade-off between signal-to-noise ratio and imaging speed, the team built a coil with 10 ladder channels around the cylinder and additional circular coils around the top, giving a total of 11 channels. For implementation of the final breast-specific MRgFUS system, they used SolidWorks CAD tools to design a flexible transducer positioner, the suspension tank, and the patient table.
The University of Utah team, headed up by UCAIR Director Dennis Parker, PhD, a professor and medical physicist in the department of radiology of the University of Utah School of Medicine, and Robert Roemer, PhD, a professor of mechanical engineering, expect to complete construction of the final device by the end of December. "We are currently in year three of our four-year funding period," Payne told medicalphysicsweb. "We will start animal testing by the end of this year and anticipate starting to treat fibroadenoma patients by summer 2011."
By Tami Freeman
Medicalphysicsweb editor
December 27, 2010
Related Reading
MRgFUS effective, safe for outpatient fibroid treatment, December 1, 2008
MRI predicts outcomes for US-guided fibroid surgery, September 25, 2008
MR signal, nonperfusion drive long-term success of MR-guided focused US therapy, May 20, 2007
ยฉ IOP Publishing Limited. Republished with permission from medicalphysicsweb, a community website covering fundamental research and emerging technologies in medical imaging and radiation therapy.