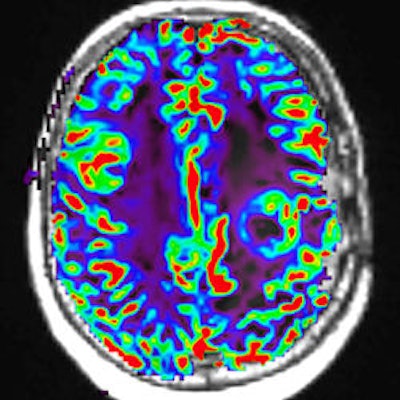
Breakthroughs are occurring in cancer treatment thanks to advances in immunotherapy, in which the body's own immune system is harnessed to attack cancer cells. The original purpose of immune cells is to identify and destroy dangerous cells. However, cancer cells have developed the ability to hide from the immune system and, therefore, uncontrolled cell proliferation occurs, leading to tumor growth.
In principle, immunotherapy makes cancer cells visible to the immune system. Therapies target the body's immune cells to identify cancer cells and also enable immune cells to destroy the dangerous cells. Therefore, immunotherapies are also called targeted therapies.
For the first time in cancer treatment, it is possible to address tumor heterogeneity in a more specific way. Immunotherapy enables us to study and manipulate the tumor microenvironment. However, to evaluate, prove, and leverage the potential of immunotherapies, it is essential to find methods that can noninvasively show changes in the tumor microenvironment.
This is where advanced medical imaging followed by computer-based analysis becomes paramount. Advanced MRI in combination with image analysis algorithms to calculate quantitative imaging biomarkers offers the unique opportunity to provide sensitive and specific tumor measurements.
The combination is especially important in clinical research studies, which aim not only to test the safety of a drug but also to assess its efficacy in certain patient population. The sensitive assessment of the therapy-induced tumor microenvironmental changes is crucial for bringing drug candidates to market.
Immunotherapy: Harnessing the immune system
Due to their inherent mechanism of action, immunotherapies are also called targeted therapies or combination therapies. The first part of the therapy is to target the immune system to attack the cancer cells systematically: The immune cells (T cells) are directed in the body toward the tumor; T cells need to be close to a cell to test if it is dangerous.
Second, immune cells are empowered to see and destroy the cancer cells. Making dangerous cancer cells visible to T cells is the task of drugs known as immune checkpoint inhibitors. T cells must be close to cancer cells to enable the checkpoint antibodies to reveal the cancer cells and fight them.
Cancer in detail: The tumor microenvironment
The tumor microenvironment of many tumor types, such as glioblastoma (GBM), the aggressive and most common form of brain cancer, is heterogeneous, meaning it is composed of different cell types.
The efficient treatment of GBM is still an unmet clinical need. Much research is focused on GBM treatment development: Multimodal therapies (including radiation, chemotherapy, and surgery) as well as exciting novel approaches such as immuno-oncology therapies are constantly being developed and tested.
The complex nature of the tumor itself requires not only more advanced therapies but also better insights and more advanced methods to prove therapy efficacy. To understand a therapy's mode of action and evaluate treatment efficacy, morphological as well as functional details of the tumor changes need to be both visualized and measured.
Immunotherapies cause different morphological and functional tumor changes than conventional therapies do. The tumor might not shrink but instead just stops growing. Complex, nonuniform tumor shrinkage has been observed. Metabolic activity can even lead to an initial increase in the size of the tumor in an effect called pseudoprogression. The key is to be able to differentiate pseudoprogression from true progression.
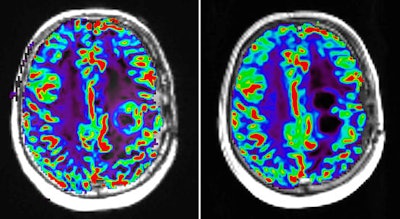
The contribution of advanced imaging
Functional MRI sequences include diffusion-weighted imaging (DWI) and dynamic contrast-enhanced (DCE) MRI. DWI measures the movement of water molecules in tissue and can be used to reconstruct neuronal pathways in the brain as well as to measure cellular density. DCE-MRI uses a gadolinium-based contrast agent that changes signal intensity and, therefore, makes blood visible in the image, which is particularly beneficial in inflammatory or necrotic tissue.
Another technique, multiparametric MRI (mpMRI), is also useful for its combination of anatomical and functional sequences within one MRI setting. This enables the assessment of functional characteristics such as tumor perfusion and cellular density alongside anatomical high-resolution lesion location.
To ensure the highest sensitivity and specify, MRI protocols must be carefully designed for each therapeutic area and clinical question, and they must be applicable in a multicenter clinical trial environment.
The impact of enhanced sensitivity on drug development
Clinical trials in cancer drug development must prove the safety and efficacy of immunotherapies in certain cancer types. This is challenging in multiple ways: finding the right patients, designing a study with adequate methods to show efficacy, and aligning with regulatory requirements, as well as finding investors for the study.
Conventional approaches in oncology clinical trials aim to show drug efficacy based on the assumption that tumor shrinkage is significant for the assessment of the change in tumor burden. Tumor shrinkage is conventionally measured using anatomical imaging modalities such as CT or MRI in combination with scoring methods based on Response Evaluation Criteria in Solid Tumors (RECIST). RECIST is based on tumor diameter, in which a reader (a board-certified radiologist) defines the disease burden by measuring the size of a lesion in an image.
Even though diameter-based methods such as RECIST have their place -- such as in late-stage clinical trials where the mode of action and drug efficacy have been understood and shown -- they are not adequate to capture complex microenvironmental changes in tumors. In particular, studies in advanced therapies, such as immunotherapy, or in heterogeneous tumors, such as glioblastoma, are prone to error when end points are used that assume tumor shrinkage.
Immunotherapy, for example, has been shown to lead to so-called pseudoprogression, the initial increase of tumor burden followed by a later treatment response. The phenomenon is called pseudoprogression because per RECIST, such tumors would be classified as progressive disease.
Recently, RECIST has been updated to RECIST1.1, with major changes in the number of lesions to be assessed and the assessment of pathological lymph nodes. However, RECIST1.1 still does not account for immunotherapy-related changes.
Several updates to RECIST that consider the potential false evaluation of pseudoprogression have emerged:
- Immune-related response criteria (irRC), which is based on criteria from the World Health Organization
- Immune-related RECIST (irRECIST), a combination of irRC and RECIST
- Immune RECIST (iRECIST), a standardized, evaluated version for use in clinical research
All RECIST amendments are valuable and useful for drug efficacy evaluation. However, they are not sensitive enough to provide more detail into therapy-induced tumor microenvironmental changes early and with scientific accuracy.
Applying less-than-adequate image analysis methods in clinical trials carries the danger of keeping cancer patients on ineffective treatments for too long, as researchers wait for a potential later response after pseudoprogression. It also wastes precious time and investment of R&D resources without getting the right answers. Besides extending the trial unnecessarily, the question is: Are the applied methods at all able to show the drug-induced changes in the tumor?
Adequate scientific methods must be applied to enable the clinical trial to reveal drug efficacy.
The insight that advanced MRI offers
CT or MRI is routinely used in the initial evaluation of tumor size, shape, and anatomic appearance. We believe that in early efficacy trials of drug candidates, it is paramount to extend the imaging protocol with sequences that allow the use of measurements (biomarkers) that adequately reflect therapy-relevant changes. Such biomarkers should guide our quantitative and objective assessment of the drug effect and overall decision about the early efficacy of novel treatments.
The most relevant sequences for immuno-oncology-related drug development are functional MRI sequences, including DCE-MRI and DWI. DCE-MRI allows for the evaluation of tumor necrosis and cell membrane permeability, and it also allows for the assessment of pharmacodynamic end points. DWI has been used to assess parameters associated with the rate and distance of water molecule diffusion that may reflect drug access as well as cellular density.
Multiple pathological studies have shown that lesions classified as pseudoprogression consist of necrotic tissue and inflammatory cells without evidence of active tumor growth or progressive disease. Pseudoprogression has been associated with significantly better overall prognosis, leading to the hypothesis that it can be considered as a marker of better prognosis and therapeutic response. Therefore, it is vital to understand as early as possible whether pseudoprogression or true progression is present.
The future of drug development
Imaging biomarkers derived from advanced MRI and calculated using image postprocessing algorithms offer a unique and powerful insight into the tumor microenvironment in vivo. Carefully designed, they can act as surrogate end points in clinical trials showing treatment-related changes in greater detail earlier than conventional diameter-based approaches.
These imaging biomarkers can aid in patient stratification, early scientific efficacy evidence, early go/no-go decisions on drug candidates, adaptive trial design, and efficient drug development financing, as well as meeting inflection points early. When used adequately in a multicenter clinical trial setting together with centralized reading, imaging biomarkers reflect therapy-related changes and reduce human error.
Diana Roettger, PhD, is head of scientific and medical affairs at IAG. Roettger is passionate about the use of medical imaging and computer-based image analysis in strategic drug development. She has more than 10 years of experience in medical imaging, algorithm design, and computer science. She holds a doctorate in computational neuroimaging and has hands-on work experience with world-leading clinical institutions. At IAG, Roettger directs the development and execution of imaging as a measure of efficacy, eligibility, and safety in drug development.
Olga Kubassova, PhD, is CEO and founder of IAG. Kubassova is a mathematician by background, with many years of effective asset positioning and implementing strategies for clinical development, growth, partnering, and exit. Her expertise is in identifying and integrating novel approaches to support fast decision-making on the efficacy of new therapeutic assets. She broadly leverages her background in computer science, mathematics, and imaging to support companies, developing novel technology platforms and decision-making systems. She has co-authored over 60 publications, books, and book chapters and is a speaker and biotech investor with a passion for improving people's health.
Both can be contacted at [email protected].
The comments and observations expressed are those of the authors and do not necessarily reflect the opinions of AuntMinnie.com.