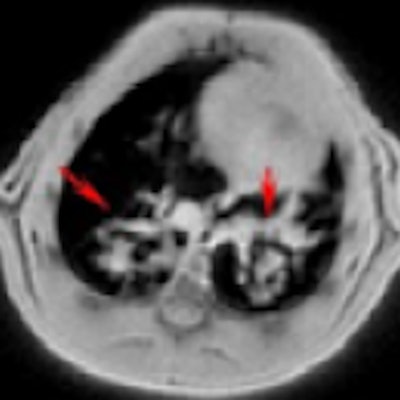
The incidence of respiratory disease is increasing throughout the world, and the demand for safe, effective drugs to tackle conditions such as asthma, chronic obstructive pulmonary disease (COPD), pneumonia, and lung cancer is rising. This growing demand has sparked more research into therapies that address the treatment of pathological lung conditions.
However, drug discovery and development are arduous and costly endeavors, with a low approval rate. Approximately nine out of every 10 drug candidates fail to win approval, a significant factor in the overall high cost of drug development.¹ While there can be numerous reasons for failure, the pharmaceutical industry benefits significantly from finding ways to more effectively evaluate drugs in preclinical studies that have a higher chance of clinical success in humans.
Effective evaluation of drug candidates
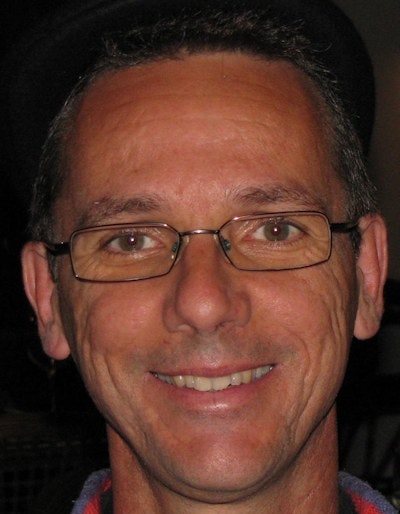
These benefits mean that the pharmaceutical industry is continually trying to find new ways to evaluate drug candidates more effectively. As such, pharmaceutical researchers can improve chances for successful drug development with an earlier and deeper understanding of drug characteristics that may impact final approval.
Improving the characterization of compounds and their effects in early and relatively noncostly phases is one way to increase the chance of success in later phases of drug development. Research on pathophysiology, and its early diagnosis and characterization, can also contribute to a better understanding of disease mechanisms and therefore increase the probability of finding an appropriate treatment.
Noninvasive preclinical lung imaging
The lung is a very intricate and interesting organ. If there is pathology in one part of the lung, the rest of the organ can compensate and the lung will continue to function. As such, standard measurements like spirometry to assess lung health are not often indicative of disease. Imaging, on the other hand, can detect early disease in these situations when conventional approaches may only show advanced disease states.
Over the past 20 years, advances in imaging technology have led to a better understanding of lung disorders and a better identification of key features for confirming diagnosis of lung disease. Researchers have found value in using noninvasive bioanalytical technologies, such as imaging, for preclinical pulmonary disease drug discovery and development.
Preclinical lung imaging methods have developed considerably, allowing examination of the lungs in small rodents at anatomical, functional, and even molecular or target levels at high spatial and temporal resolution. These methods have become invaluable in preclinical pharmaceutical research. The development of imaging strategies that meet the requirements for use in a clinical setting may facilitate the translation from animal models to human subjects because they minimize changes in experimental paradigms while the model organism is changed.
Enhancing animal welfare
Biologically relevant small animal models have become important tools for the study of the fundamental aspects of human system function and dysfunction. In preclinical research, which encompasses all endeavors before testing compounds in humans, a significant portion of the activities is performed in small rodents, including mice. The main reasons for using mice are the possibility of using genetically altered animals and the availability of several disease models in this species. Additionally, smaller amounts of compound are needed for in vivo drug testing in mice than in higher species.
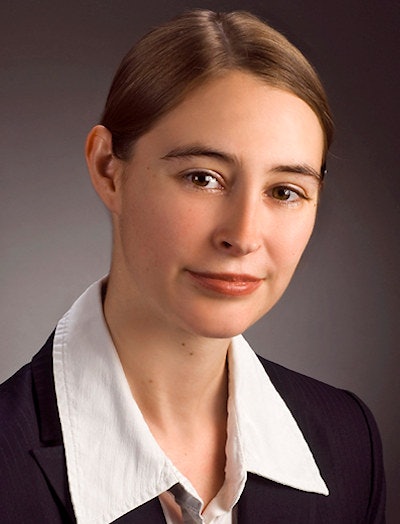
Imaging technology can support the replacement, reduction, and refinement of animal studies, otherwise known as the 3Rs.² For example, traditional methods of investigating lung disease in animal models, such as bronchoalveolar lavage (BAL) analysis and histology, often rely on invasive handling, restraint, or even euthanasia of the animal, followed by sampling and measurement of body fluids and tissues. However, noninvasive imaging techniques that provide images of body systems are readily available to use as alternatives, even for small animals such as rodents.
With the use of imaging, toxicological effects of compounds on structure or function of organs can also be studied in vivo without tissue dissection, sectioning, and staining. As a result, these in vivo assessments can eliminate the need to sacrifice the animal. Additionally, noninvasive imaging may considerably simplify the assessment of compound efficacy in small rodents, as the animal can be used as its own control, limiting any intrasubject variability and greatly improving the statistical power of results.
Imaging techniques
Lung imaging approaches have provided health professionals with a better understanding of how the lung is affected by a range of disorders so treatments can be optimized. The use of imaging techniques in preclinical studies has enhanced understanding of disease mechanisms by observing changes at systemic, organ, tissue, cell, and molecular level, in response to different physiological or environmental conditions and disease states, under prescribed experimental conditions.
Today, cost-effective and high-throughput longitudinal study of animal models can be achieved using imaging techniques such as MRI, CT, and PET.
The principal strengths of MRI are its noninvasiveness, high spatial resolution, and excellent soft-tissue contrasting capabilities. It is a very flexible technique that allows one to analyze from the brain to the limbs and to address a multitude of diseases. Because of its noninvasive nature, imaging technology like MRI enables one to repeat measurements on the same subject without harm. This provides the basis for the use of MRI in preclinical drug development. Another fundamental aspect is MRI's ability to translate preclinical research to clinical practice by imaging both small animals and humans.
MRI is a particularly attractive imaging option for the evaluation of animal models since it provides high spatial resolution combined with excellent contrast to distinguish between normal and pathological tissue. In addition to providing anatomical information, MRI can perform functional evaluation, both of which are especially valuable for noninvasive animal studies.
CT is considered the gold standard for clinical lung imaging, and micro-CT provides x-ray imaging in 3D by the same method used in hospital CT scans but on a small scale with significantly increased resolution. Micro-CT systems providing high-resolution images and rapid data acquisition are a cost-effective means for detecting tissue structures, skeletal abnormalities, and tumors in small animals.
Micro-CT can be used to supplement data from other molecular imaging techniques by providing images of the very fine scale internal structure of objects nondestructively. It can generate 3D images of a sample's morphology and internal microstructure with resolution down to the micron level. Strategies to reduce radiation burden while keeping the spatial resolution have rendered the technique useful for repetitive, longitudinal measurements in the same subject.
PET detects radiation emitted from tracer substances injected into the body and labeled with positron-emitting isotopes. These isotopes are bound to a targeted tracer. For example, the most prominent tracer, F-18 FDG, combines F-18-labeled fluorine with a type of glucose to target, in particular, cancer cells. PET detects gamma rays generated at the target site when a positron emitted from the tracer is captured and annihilated by an electron in the tissue.
As one of the most sensitive imaging approaches with picomolar amounts of radiolabel being readily detected and quantified in vivo, PET is the method of choice for pharmacokinetic studies of biologically active compounds, for instance, drugs or drug candidates.
Multimodality
These imaging techniques are complementary, each offering its own strength of sensitivity. Anatomical modalities such as CT and MRI mainly reveal the structure of the tissues and organs, while the functional PET visualizes the physiology and function of the tissue.
For example, MRI and CT, with their high spatial resolution, provide a good anatomical reference for molecular data obtained with high sensitivity PET. This might be achieved by postprocessing of data obtained in different imaging sessions or by simultaneous multimodality small animal imaging such as PET/MRI or PET/CT.
Pharmaceutical companies rely on understanding the biology underlying the disease process to develop compounds or therapies. For the lungs specifically, that requires inducing a disease status like lung inflammation. For example, MRI can detect inflammation in the lung very clearly. So researchers elicit the disease status, quantify it with MRI, and then administer the compound to assess its efficacy also using MRI. Standard techniques such as histology would not detect the dynamics of the compound, but MRI can. That enables researchers to get a compound into clinical trials, and eventually to market, much faster.
Technology has also made important leaps for data reconstruction and analysis, and better instrument stability has improved the reproducibility of experiments. More specifically, access to ultrashort echo time acquisition (UTE) for MRI experiments was an important development that now enables researchers to analyze difficult tissues with more traditional techniques.
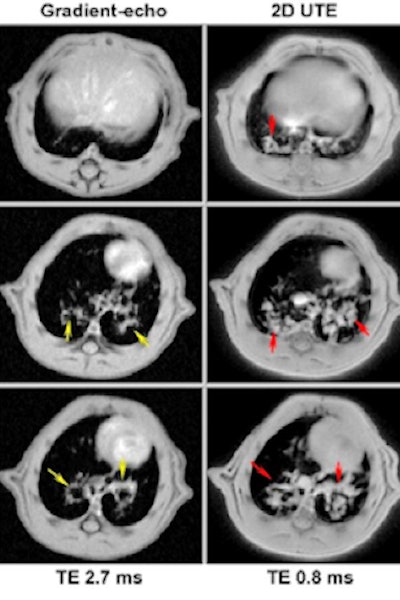
Ensuring viable and safe drug candidates
Preclinical imaging techniques are central to evaluating the effectiveness and safety of new treatments and describing drug distribution patterns before clinical use. Preclinical small animal imaging has provided valuable insights into the mechanisms of lung disease and the effects of treatment. Some imaging techniques like MRI also can be used to observe organs without euthanizing the animals -- reducing the numbers of animals required in the experiments, thus making them both more humane and more cost-effective.
Innovation in imaging technology has helped researchers to better understand the underlying causes of many lung disorders. Such research has identified key features for confirming the diagnosis of lung disorders, such as pneumonia, pulmonary embolism, or pulmonary hypertension; detecting lung tumors; and staging bronchial carcinoma. This knowledge informs the development of novel therapeutic strategies, enabling pharmaceutical companies to evaluate drug candidates more efficiently -- efforts that ultimately can improve patient outcomes and save lives.
References
1. Counting the cost of failure in drug development. Pharmaceutical Technology. https://www.pharmaceutical-technology.com/features/featurecounting-the-cost-of-failure-in-drug-development-5813046/. June 19, 2017.
2. Beckmann N, Ledermann B. Noninvasive small rodent imaging: Significance for the 3R principles. In: Kiessling F, Pichler B, Hauff P, eds. Small Animal Imaging. Springer, Cham; 2017:69-87.
Nicolau Beckmann is has an MSc in physics from the University of São Paulo in Brazil and a PhD in biophysics from the University of Basel in Switzerland. Currently, he is the head of an imaging and histology group at the Novartis Institutes for BioMedical Research in Basel and an assistant professor in biophysics at the University of Basel.
Sarah-Rebecca Herrmann is a product specialist, MRI, at Bruker BioSpin.
Bruker is enabling scientists to make breakthrough discoveries and develop new applications that improve the quality of human life. Bruker's high-performance scientific instruments and high-value analytical and diagnostic solutions enable scientists to explore life and materials at molecular, cellular, and microscopic levels. In close cooperation with customers, Bruker is enabling innovation, improved productivity, and customer success in life science molecular research, applied and pharmaceutical applications, microscopy and nanoanalysis, and industrial applications, as well as in cell biology, preclinical imaging, clinical phenomics, and proteomics research and clinical microbiology.
The comments and observations expressed are those of the author and do not necessarily reflect the opinions of Auntminnie.com.